
Champion of Regeneration among Mammals
The word ‘regeneration’ sounds truly fascinating when it comes to restoring a lost body part or organ. An image of the epic Serpent Gorynych of Russian fairy-tails immediately comes to mind, a magical many-headed beast that could easily regrow its lost heads. Another example, from our reality, is the salamander, the all-round champion of regeneration among animals, which is able to restore not only its limbs or tail but also eyes and even its heart. Humans, as well as other warm-blooded creatures, can only dream of that.
However, even the class of mammals, which generally have extremely low regenerative abilities, contains exceptions. One such outlier is the spiny mouse, a small rodent that is able not only to heal huge wounds on its skin but also restore its spinal cord after a rupture. Amazing, isn’t it? But how does this animal do that? And why are other mammals incapable of dealing with injury as effectively? Can humans possibly “acquire” such a superpower from spiny mice? This review article written by a young Russian researcher presents answers to these questions
What is regeneration? Strictly speaking, restoration of damaged tissues and organs can be classified into repair and regeneration. The first process, leading to the formation of a scar at the site of injury, ensures the integrity of the organ rather than its functions. Regeneration, on the contrary, enables an almost complete restoration of both the original architecture and function of the organ.
Most mammals, humans including, have very limited regenerative abilities. Recovery in patients with extensive injuries or after surgery usually takes the path of repair; i. e., a “patch” of connective tissue cells and disorganized intercellular material emerges at the site of injury, which leads to a loss of function in this area and often causes chronic pain.
By studying animals with remarkable regenerative abilities, science could find clues as to how humans, too, may achieve “proper” healing of bodily damage.
Up the down staircase
When we “climb up” the evolutionary tree and trace the ability to regenerate, we find out that the more complex the organism, the poorer its regenerative abilities (Brewer, 2018). Some of the most primitive multicellular creatures – sponges – can literally reassemble themselves even if crushed into individual cells. And many invertebrates, such as worms, jellyfish, corals, and echinoderms, are able to regenerate the organism from a body fragment.
Also widely known is the ability to regenerate limbs and tails in amphibians. Yet the higher up the evolutionary tree, the worse the situation. Why is that? Why does this property, which seems to immensely benefit natural selection, diminish during evolution? The reasons are unclear. The question remains open whether these limitations contribute to adaptation or whether they randomly took hold in different evolutionary branches.
In fact, the regenerative abilities of vertebrates are not too bad. Arguably, all of them – from fish to birds and mammals – are able to regenerate. But here is a catch – most of them can only do that in the early stages of development.
A striking example of the loss of regenerative abilities with age is the restoration of finger phalanges in humans. It is known that in children up to about 12 years of age, amputated terminal phalanges can grow back together with the nail plate, a phenomenon impossible in adults. The exact reasons why the ability to regenerate diminishes with time remains to be determined.
Among vertebrates, fish and amphibians regenerate best in adulthood. For example, tailed amphibians (salamanders and newts) can regrow limbs and restore internal organs. They can regenerate their nervous system. They can even “remake” the lens of the eye, which is why they have become the favorite object of research for regenerative biologists (Song et al., 2010).
Widely known is the tail regeneration phenomenon in reptiles, which can regrow a tail after voluntary discarding it (autotomy) as an act of protection from predators. This is not full-fledged regeneration, however, since the newly grown tail will have neither “normal” innervation nor real vertebrae, which will be replaced by an elongated cartilaginous tube.
Speaking about humans, even our distance ancestors knew about the liver regeneration possibilities in adults – recall the myth of Prometheus and the Eagle of Zeus. The restoration of this organ occurs through the so-called compensatory hypertrophy, i. e., through an increase in the number or volume of cells. Unfortunately, the other internal organs cannot claim such ability.
Nevertheless, some mammals – very few – show truly extraordinary abilities for regeneration. One such example is house mice of the MRL/MpJ laboratory line and related lines. These animals are able to restore penetrating damage to the auricle as well as skin, joints, and the cornea of the eye. Interestingly, this ability arose spontaneously in them; initially, it did not serve as a selection criterion when creating the line. Possible causes include changes in cell cycle regulation and a weaker inflammatory response to injury (Heydemann, 2012).
However, even the outstanding talents of these linear mice pale beside the champions of regeneration among mammals, i. e., spiny mice.
It all began with the tail
Spiny mice have been our laboratory animals and pets since the beginning of the twentieth century, which is not surprising as these rodents are active and curious and they easily get along with the people who care for them. However, their amazing regenerative abilities were noticed only a few years ago.
It all began with the discovery of a very unusual property in spiny mice, i. e., autotomy, or discarding a bodily part as a defensive reaction (Seifert et al., 2012). Aautotomy is very rare phenomenon in vertebrates, and it usually manifests itself in the casting of the tail with a fracture of the vertebrae (true autotomy, as in lizards) or loss of skin on the tail (false autotomy, as in jerboas and dormice) (Dubost and Gasc, 1987).
Spiny mice, too, can shed their tails, but unlike reptiles, they do not regenerate them, so picking up these animals by the tail, as is done with ordinary mice, is a bad idea (Shargal et al., 1999). The autotomy of spiny mice involves the discarding of flaps of back skin, which itself is very fragile and can easily tear off if someone grabs the animal. Bleeding from this type of injury is minimal (Pinheiro et al., 2018).
No special zones were found in the mice’s skin where it could peel off as in the case of skin shedding by geckos and skinks (Seifert et al., 2012). The skin fragility is due to special properties of the intercellular matrix: on the one hand, it is infirm, allowing for skin flaps to easily drop off; on the other hand, it promotes healing at the site of injury. Epithelium quickly covers the wound surface, a process followed by a complete restoration of hair follicles, glands, muscle layer, and other skin components.
As a result, the injured area completely regenerates without forming a scar. Moreover, the recovery process itself seems not to bother the animal at all – it continues moving and eating as usual (Seifert et al., 2012).
Firstly, spiny mice are semidesert animals adapted to living in a dry climate and on low-nutrient food. Due to the conservation of moisture, they secrete very concentrated urine, with the urea level reaching 4.8 m/l, one of the highest figures in mammals. Therefore, studies on spiny mice are used in the field of urinary system physiology (Shkolnik and Borut, 1969).
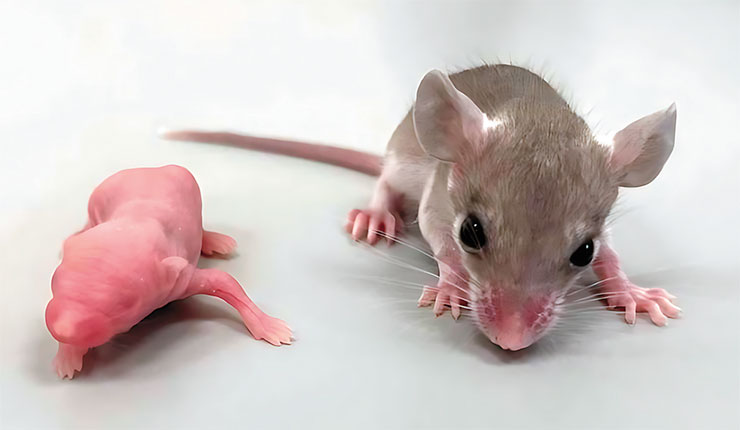
The organism of these small mammals stands out for its highly efficient use of nutrients. Their metabolic rate is 25–30 % lower than expected for their size, and in the species A. russatus, it is one of the lowest among rodents (Degen, 2013).
Another consequence of living on scanty food is a decreased level of insulin secretion in response to meals (Rabinovitch et al., 1975). As a result, in captivity, given a free access to fatty, high-calorie foods, spiny mice are prone to developing obesity. Furthermore, on such a diet, the animals develop hyperglycemia and hyperplasia of Langerhans islet beta cells, which subsequently degenerate. In other words, they develop type 2 diabetes mellitus, which is why the spiny mouse presents a convenient animal model of this pathology (Shafrir et al., 2006).
Spiny mice have another amazing feature – they are the only rodents whose females menstruate. At the end of the 11‑day estrous cycle, they experience shedding of the endometrium (the inner layer of the uterus), accompanied by bleeding. This fact opens up the possibility of using spiny mice as a model to study reproductive biology in human females (Bellofiore et al., 2018).
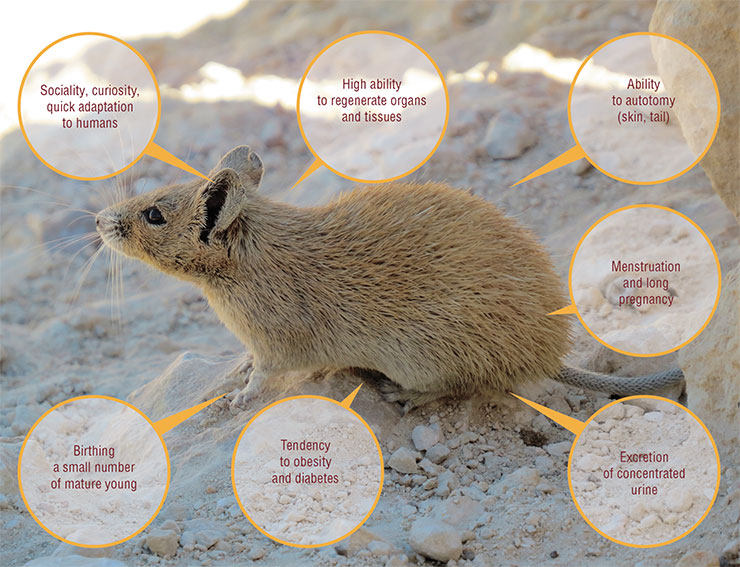
Their pregnancy lasts 39 days, which is noticeably longer than in other rodents. The mousekins are born covered with fur, with their eyes and ear canals open. They have sufficiently developed brains; soon after birth, they are able to eat solid food and may leave the nest, unlike baby mice and rats, which are unable to move independently after birth. Therefore, spiny mice are a convenient object of research for studying brain development and neuroplasticity as well as in behavioral science (D’Udine and Alleva, 1988; Cohen et al., 2010)
To elucidate the molecular foundations of this phenomenon, scientists analyzed the proteome (the complete set of proteins produced) and transcriptome (the complete set of RNA molecules read from DNA, including protein “matrices” and regulatory RNAs) at lesion sites in spiny and house mice. The analyses revealed substantial differences in gene activity between the species (Brant et al., 2015; Simkin and Seifert, 2018).
In comparison with house mice, the site of injury in spiny mice is characterized by a reduced level of inflammation-provoking molecular factors such as cytokines Il6, Cxcl3, Ccl12, Ccl7, or Il1b. Moreover, the composition of immune cells differs too; i. e., anti-inflammatory M2 macrophages are present in large quantities while their “antipode” M1 macrophages and neutrophils are few in number. This is evidence of a unique immune response at the site of injury (Brant et al., 2015).
The proteome and transcriptome analysis also showed that skin damage in spiny mice results in the upregulation of genes associated with muscle development during embryogenesis. Thus, the restoration of subcutaneous muscles probably follows the same path as during their development in the fetus. Animals also displayed active synthesis of enzymes, such as matrix metalloproteinases involved in the restructuring of intercellular substance, and reduced production of type I and III collagens associated with scar formation (Gawriluk et al., 2016).
When the brain is screwed…
Following the discovery of autotomy, it soon became clear that the ability of spiny mice to heal large lesions was not limited to the skin. In the course of studies, the animals were injured in various ways: by piercing the auricles, injecting toxins into the muscles, simulating myocardial infarction, and inducing acute and chronic kidney damage. The picture was always the same – spiny mice coped brilliantly with all kinds of damage!
Of particular interest, from theoretical and practical perspectives, is the ability of spiny mice to regenerate the nervous system. Mammals are already the worst of the worst among vertebrates in terms of regenerative abilities but their nervous system is the worst in this respect among all the systems and organs. Thus, in humans, damage to the central nervous system often leads to irreversible loss of its functions; today about 80 million people worldwide live with disabilities due to brain or spinal cord injuries (Sofroniew, 2014).
A trauma to the central nervous system usually leads to the development of an astrocytic scar, formed by astrocytes, i. e., the glial (“maintenance”) cells of the brain. On the one hand, this is a protective reaction aimed to support the remaining nerve cells; on the other hand, this is an obstacle that impedes the growth of axons (long neuron processes that conduct nerve impulses) and prevents the restoration of nervous tissue functions (Sofroniew, 2014).
Hereditary information actualizes in a living organism from the DNA to RNA and from the RNA to proteins. The hierarchy, or linkage, of disciplines is built accordingly: genomics studies the genome and genes; transcriptomics looks into the synthesis and distribution of transcripts (RNA molecules); proteomics deals with the set of protein molecules; and metabolomics studies the set of all metabolites formed as a result of biochemical reactions
Since spiny mice form no scars during skin regeneration, a suggestion was put forth that they develop no astrocytic scars either. This idea was confirmed when studying spinal cord injuries in these animals (Streeter et al., 2020).
It turns out that spiny mice exhibit a unique pattern of gene activity in astrocytes and connective tissue cells (fibroblasts) in response to a spinal cord injury. The composition of the intercellular matrix at the site of injury in spiny mice also differs markedly from house mice, with a higher content of components that ensure the growth and maintenance of new axons and a lower content of those that interfere with these processes.
The former components include keratan sulfates, i. e., sulfated polysaccharides found in bone and cartilage tissue and in the cornea of the eye. In the brain, these chemical compounds participate in regulating the cellular cytoskeleton growth and maintaining the cell shape, in the transport and storage of neurotransmitters, in the growth of axons, and in the formation of new synapses.
The site of spinal cord injury in spiny mice is characterized by a sharp increase in the content of the b3gnt7 enzyme, which is essential for the synthesis of keratan sulfates. Scientists posed a question whether this enzyme could be “tamed” to work in other organisms, facilitating the growth of new axons and the healing of spinal injury. The answer turned out to be positive, and the first steps have been made on this path.
The B3GNT7 gene, which encodes this enzyme, was made to work in CHO cells (a culture of Chinese hamster ovary cells). Human neurons that were cultured on a “substrate” of these cells showed a vigorous growth of processes, including axons (Nogueira-Rodrigues et al., 2022). So the B3GNT7 gene could theoretically serve to treat spinal injury.
The good news is that humans, too, have this gene, but one has yet to find a way how to make it work more actively than usual. To this end, one can deliver additional copies of the B3GNT7 gene to the site of injury using genetic vectors, e. g., adeno-associated viruses. The work of these “extra” genes will ensure the growth of new axons and restoration of spinal cord functions. So the discovery of spiny mouse genes responsible for spinal cord regeneration may lead to the development of a fundamentally new approach to the treatment of spinal injuries, i. e., gene therapy.
Brain restoration, too, is of great interest. A source of renewal of lost brain cells may be associated with neuronal stem cells, which give rise to both neurons and glial cells. In adult mammals, these cell precursors are found in two zones located in the hippocampus and the lateral wall of the brain ventricles.
It turned out that spiny mice have several times more stem and dividing cells in these zones, compared to house mice. Such a large number of neuronal stem cells may indicate that these rodents also have increased regenerative abilities when it comes to the brain, which opens up a wide field for research (Maden et al., 2021).
Today we know that spiny mice can effectively regenerate after extensive damage to their skin, heart, kidneys, skeletal muscle, or spinal cord.
Unique immune reactions, a peculiar structure of the intercellular matrix, and the inclusion of “embryonic” genes – these are apparently the main properties enabling spiny mice to easily cope even with severe injuries. In this respect, these animals are of great interest for regenerative medicine.
An in-depth look into molecular mechanisms underlying regeneration in these animals will help identify genes and signaling pathways that can serve as targets in the treatment of various injuries and will ultimately enable the design of revolutionary methods for treating injury in humans. After all, the very existence of mammals that have reclaimed the ability to regenerate in the course of evolution presents evidence of absence of a fundamental ban on this ability in other highly organized animals. Including you and me.
References
Modestov A. and Kislova А. Regeneration at fingertips // Biomolekula. 2021. URL: https://biomolecula.ru/articles/regeneratsiia-na-konchikakh-paltsev [in Russian].
Tereshina M. B., Arslanova K. R., Volkov S. A., etc. Big brain in exchange to regeneration // Neirotekhnologii budushchego (Neurotechnologies of the Future): Abstracts Conf. “Neirokampus 2022: start! (Neurocampus 2022: Start!)” (Sept. 4–7, 2022.). 2022.N 1. P. 67–69 [in Russian].
Haughton C. L., Gawriluk T. R., and Seifert A. W. The Biology and Husbandry of the African Spiny Mouse (Acomys cahirinus) and the Research Uses of a Laboratory Colony // J. Am. Assoc. Lab. Anim. Sci. 2016. V. 55 (1). P. 9–17.
Maden M. and Varholick J. A. Model systems for regeneration: the spiny mouse, Acomys cahirinus // Development. 2020. V. 147 (4). dev167718.
This publication is based on the article published at the Biomolecula portal (biomolecula.ru) as part of 2022/2023 Bio/Mol/Text Competition
The author thanks her scientific supervisor G. A. Nosov, Cand. Sci. (Biol.), staff member of the Federal Center for Brain and Neurotechnology, FMBA Russia, for profound and continued support
