
Ground Zero: Megaquakes Pose Main Threat to Coastal Safety
The problem of assessing the tsunami hazard of underwater earthquakes sets a major task for seismology research in the Pacific region, where most powerful (with a magnitude of up to 9.0) earthquakes occur in the surrounding subduction zones. The damage caused by tsunamis intensifies through the suddenness and quickness of these events, the havoc they wreck, and the high probability of human fatalities in the impact zone. Since the sea level experiences constant disturbances, caused by wind waves, storms, and tides, the height of tsunamis has some natural threshold below which these waves escape observation and above which—immediately become dangerous. A challenge for creating effective protection from this natural disaster comes from the fact that tsunamis very rarely hit a specific area of the coastline. Even in the most tsunami-active areas of the Pacific Ocean (such as Japan, Chile, or Peru), strong tsunamis with fatalities occur once in 30–50 years, and catastrophic events, once in 100–150 years. These periods far exceed the frequency, e.g., of hurricanes and floods, and are comparable with that of earthquakes and volcanic eruptions. In terms of fatalities and total damage, tsunamis rank fourth among natural disasters and catastrophes, after earthquakes, floods, and typhoons. The world statistics on natural disasters in the 20th century, which claimed more than four million lives, shows that tsunamis account for a relatively small percentage of fatalities, just over 1%. The Indonesian disaster of 2004, which claimed 228,000 lives, immediately raised this share to 20%
The early morning on a Sunday, December 26, 2004, on the northwest coast of Sumatra was sunny and hot. Women in a village of Lhoknga, where houses hid among coconut palms growing right near the sandy beach, had fed their chickens and were about to wake up their kids for breakfast. The clock showed 7:58, when the earth suddenly trembled and glassware set off clinking. The first shock was followed by strong vibrations, swinging trees and walls, accompanied by a low, aggravating roar. Strong shocks continued for almost five minutes; then the earth seemed to calm down, sometimes shuddering from the past cataclysm. The quake frightened people in this part of the island—most of them had never experienced so heavy shocks. But there were neither victims nor much destruction. The ocean, too, remained quiet. No warnings or alerts came from the authorities. But in 15 minutes, on the western horizon, where the deep blue waters met the light-blue sky, a dark line appeared, rapidly approaching the shore. A growing noise came from the ocean. When the villagers noticed it and saw a wave approaching the shore, it was too late to run. Although the hills that could have saved them lay at no more than 500 meters, the torrent that rose when the first wave rolled onto the beach moved too fast, carrying torn-out trees and house wreckage. The people caught up in it had virtually no chances of survival. Several hundred people who lived in that Sumatran village, which happened to be closest to the source of the earthquake, became the first victims of the devastating tsunami, which hit the news worldwide that day. In the next few hours, the tsunami claimed another 228,000 lives in 15 countries on the coast of the Indian Ocean.
No one saw it coming: Indonesia, December 26, 2004
Twelve kilometers east of Lhoknga, behind the cape where the Sumatran coast bends east, in a bay facing not the great Indian Ocean but the marginal Andaman Sea, there was a city of Banda Aceh with a population of about 200,000 people, the capital of the Aceh province. Its long history, dating back to the 15th century, gives no evidence that the city had ever experienced any tsunami attacks, but now its name has become synonymous with the Indonesian tragedy of 2004. It was here that one took those dramatic shots, unbelievable even for specialists, which captured a torrent of what was not even water but some unthinkable mixture of sand, stones, tree trunks, fragments of buildings, pieces of furniture, wrecked cars, etc., that tore through the streets without stopping. This torrent carried what had recently been urban areas adjoining the northern, seaport district of the city. The tsunami wave wrecked these areas to the ground; photos from there resemble those of Hiroshima after the atomic bombing. No one had ever warned the people of Banda Aceh about the tsunami hazard, but even if warnings had been issued, the population of this area with predominantly small, single-storey houses would have had nowhere to escape.
Having destroyed Banda Aceh, the tsunami wave continued to move eastward, through the Strait of Malacca to the coast of Malaysia and Thailand. There, it first reached the Thai island of Phuket, whose hotels—it was Christmas time!—teemed with tourists from Europe. The shocks from the earthquake itself were weak there, of magnitude 3 to 4, so that not all people outside the buildings noticed them. The small retreat of the sea from the coast prior to the first wave alerted no one either. People were lying on the cool morning sand; kids were playing in the small, rolling waves. A white breaking wave several meters high that appeared in the distance did draw attention to itself, but only a few of those who saw it managed to escape: only those who had been the first to realize the danger managed to reach the hotel and rush up to the second or third floor. Over the next 10—15 minutes, several thousand tourists drowned in the streams of seawater that flooded the Thai beaches.
In the Indian Ocean itself, the tsunami waves destroyed almost all the settlements on the northwestern coast of Sumatra and continued to move westward, at a great speed, towards Sri Lanka, India, and the Maldives islands. Indian seismic stations recorded an earthquake; on-duty seismologists determined its location and approximate magnitude; however, their procedures envisaged no further action to assess tsunami hazard. Here, it should be noted that throughout the 20th century, the west coast of India had experienced only one dangerous tsunami, almost 60 years prior to this tragic event, in 1945, which occurred after an 8.3 magnitude earthquake on the border with Pakistan. There had been no tsunami fatalities in contemporary history on the east coast of India and in Sri Lanka. The 2004 tsunami killed 30,000 people in Sri Lanka and almost 11,000 in India. The last victim of this tsunami was a man who drowned on one of the beaches in Kenya, where the tsunami waves, still three meters high, arrived eight hours after the earthquake.
In total, people from 55 nations lost their lives due to this natural calamity, which directly affected the coast of 15 states of the Indian Ocean Basin. For some of these nations, e. g., for Sweden (544 victims) or Finland (176 victims), these fatalities represented the greatest loss from natural disasters in the entire history of these countries.
The Operations Center for the International Tsunami Warning System in Hawaii immediately began processing data for this event. The center’s first bulletin, reporting a strong earthquake of magnitude 8.0 in the Sumatra region, came out 15 minutes after the start of registration of the earthquake. The subsequent bulletins raised the magnitude to 8.5. Importantly, magnitude 9.0 reports appeared only on the next day, when US seismologists collected and processed records from superlong-period instruments that registered the Earth’s own oscillations caused by the earthquake. Nevertheless, even magnitude 8.0 far exceeds the threshold value for declaring a tsunami alarm, at least for the nearest coast. India, Indonesia, and other countries of the Indian Ocean Basin failed to alert the population because they had no national tsunami warning systems at that time. Consequently, these countries had no decision-making procedures about alarms and, most importantly, no system for communicating information to the public. Unless such a system, as well as protection plans, exists, all warnings of an impending catastrophe communicated via any channels to embassies, consulates, and military and civilian authorities in the affected areas would be ineffective.
Megatsunami instead of tsunami: Japan, March 11, 2011
Seven years later, not only seismologists, the whole world shivered at a stream of urgent messages from Japan, where a massive earthquake occurred on March 11, 2011, followed by a devastating tsunami. The earthquake began at 14:11 local time, at the height of a working day (Friday); its epicenter was located in the ocean, about 150 km off the east coast of Honshu. The earthquake source was a fault line along the main lithospheric interface between the oceanic crust, moving gradually underneath the chain of Japanese islands, and the overlapping protrusion of the Japanese microplate. The displacements along this fault line reached 30—50 m; some reports even give the incredible, never-before-measured 60—80 m. The transverse length of the fault line was 150 km; it extended 450—500 km along the island arc. Coastal GPS stations recorded a horizontal displacement of the eastern coast of Honshu by 5 m and a lowering of the coast by 1—1.5 m. The earthquake caused substantial deformations of the seabed over an area of more than 15,000 km2, which resulted in the most devastating tsunami in the Japanese history.
This coast of Japan, known as Tohoku, hosts dozens of memorials to tsunami victims of past centuries, which have seen many destructive events, killing thousands of people (e. g., the Sanriku tsunami killed 27,122 people in 1896). However, the scale of the disaster that struck this coast on March 11, 2011 surpassed everything ever known. The population and the authorities were clearly unprepared, despite the costly programs for counteracting natural disasters that had been carried out over the past decades.
The Japan Meteorological Agency (JMA) in Tokyo, whose functions include urgent population alerts about earthquakes and operational tsunami forecasting, immediately began processing information from 1200 seismic and 200 tide gauge stations that recorded the earthquake and the ensuing tsunami. As early as 4.5 s after the first registration of oscillations by the nearest seismic stations, an early-warning message appeared on all television channels: “Major earthquake is occurring!” As early as in 2 min 40 s, the JMA issued the first magnitude estimate (7.9) and a tsunami forecast (up to 6 m). The alert was followed by a stream of urgent messages with an ever-increasing magnitude and expanding zones for tsunami alarm and expected tsunami heights.
Unlike Indonesia, which had no national tsunami warning system in 2004, Japan was best prepared among all countries in the Pacific Basin, both technically and organizationally. In terms of speed, the JMA urgent reporting service performed above its own standards, literally at the limit of technical capabilities. Nevertheless, the outcome was 15,884 dead and missing and over a quarter trillion dollars in material damage. The authorities and population of the Tohoku region were prepared to face a tsunami, but not of that power and scale. This event shows the urgency to realize that extremely rare megaevents pose real danger and incorporate hazard assessment for such events into the overall strategy for protection against natural disasters.
Although this region had a very dense geophysical observation network, no short-term (months, weeks, or days) earthquake precursors had been reported. Meanwhile, geologists who studied traces of paleoevents (prehistoric earthquakes and tsunamis) in this part of Japan pointed out in their publications that the AD 869 Jogan earthquake, known from the chronicles, caused a catastrophic tsunami that reached 5—7 km deep inland on the Sendai plain, which in itself showed the scale of the event. Among these scientists were Koji Minoura, Professor of Geology at Tohoku University, and professional geologists who worked for the TEPKO Corporation, which owned the Fukushima–Daiichi nuclear power plant, most severely affected by the tsunami. However, neither the government nor the corporate leadership paid attention to their warnings that such a devastating earthquake and tsunami might happen again in the Tohoku region. This short-sightedness resulted in a severe accident at the plant, leading to large-scale radioactive contamination and complete evacuation of people from a 30-km zone, a formidable endeavor in the overpopulated Japan.
Severo-Kurilsk Tragedy, November 5, 1952
All the Kuril Islands lie in a seismic hazard zone; the ground here shakes quite often, and even short-time visitors quickly get used to it. However, the tremors that began early in the morning on November 5, 1952 immediately surpassed everything that the people of the town of Severo-Kurilsk on Paramushir Island, the largest of the North Kuril Islands, had ever experienced. They woke up from strong shocks and in the darkness—the local power station did not work at night—quickly dressed their kids to take them outside of buildings. The ground went rocking and rolling, wooden barracks cracking; glass window panes collapsed; furnaces crashed inside. Most people were unaware that a tsunami could follow the earthquake because they had only recently settled on the Kuril Islands, shortly after the Japanese troops were withdrawn from them in 1945. Thirty minutes after the earthquake, people heard a distant rumble coming from the ocean, and a minute later, the first wave, 5—7 m high, fell down on the coast. The military were the first to see it coming through the strait between the islands of Paramushir and Shumshu; they started firing their guns into the air, trying to warn other people. The wave flooded to a half the houses closest to the shore and ebbed away, carrying a lot of human bodies and debris. The people had barely recovered from the horrors they had been through, when in 15—20 minutes, another, even higher wave struck the town. With a height of more than 10 m on the edge, it crashed everything on its way; only the foundations of houses survived. The water walloped so hard that heavy machines and steam winches attached to concrete foundations went off and bounced far away. The wave turned over and dragged along heavy tractors and huge all-terrain tracked vehicles. The torrent tore through the town to the surrounding sopki-hills, where people had run after the first wave, and rolled back into the valley where the central part of the town was located, creating a giant whirlpool, where house wreckage swarmed together with people clinging to logs and small vessels. “Houses and roofs were thrown off like matchboxes and carried into the sea,” wrote one of the witnesses. The strait between the islands teemed with floating houses, roofs, and other debris, on which people tried to escape. The air temperature was about 0 °C (the first snow had fallen the day before); the water temperature was 6—8 °C.
The Kamchatka earthquake and tsunami on November 4, 1952 has been the largest natural disaster on the territory of Russia throughout its modern history, both in the energy release from the Earth’s interior and in the scale of destruction and number of fatalities. The town of Severo-Kurilsk was completely destroyed. The exact number of tsunami casualties may have been counted but was never made public. During the perestroika, a figure appeared for the civilian fatalities—2336 people. However, most of the people who lived back then in the Kuril Islands served in the army. The book The Time of the Red Fish by the Kamchatka journalist and ethnographer A. A. Smyshlyaev gives an expert estimate for the total number of fatalities in the North Kuriles—from 15,000 to 17,000 people.
The last tsunami comparable in strength with the 1952 event occurred in Kamchatka in 1737. We know about from the detailed accounts by Stepan Krasheninnikov, a member of the Second Kamchatka Expedition, who arrived in Kamchatka from Okhotsk two weeks after the earthquake.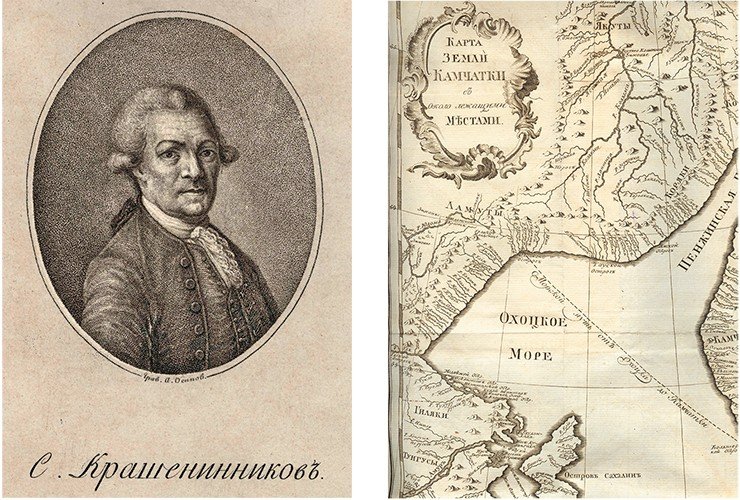
From Krasheninnikov’s report to Profs. Johann G. Gmelin and Gerhard F. Müller on November 14, 1737:
“On the 6th day of October [October 17 N.S.] at 3 o’clock past midnight, the earth shook so violently that many booths fell down and people could not stand, and it lasted for a quarter of an hour. This shake came in waves from south-southeast, and after the waves, the earth shook for a long time, and when the shaking stopped, waters came suddenly from the sea with a great noise, rising as high as three sazhens [6.3 meters], and went at once far back into the sea.
“When the water ebbed away, the earth shook again, this time only slightly, and then again water came from the sea to the same place where it was the first time. Just like the first water, it left without staying back into the sea so far that in the strait, which lies between the first and the other island [the Second Kuril Strait], a great wild stone appeared a sazhen above water. After a quarter of an hour, for the third time, water came from the sea, with a great noise, ten sazhens [21 m] above ground, and carried away many dwellings of the natives. This water ran off into the sea again, without staying, and having reached the sea, it came and went for two days.”
Seismological catalogs of that time assigned that earthquake only an 8.5 magnitude on the Ms scale. But modern estimates, based, inter alia, on instrument records, put it at 9.0 on the longer-period Mw magnitude scale, which more accurately reflects the intensity of the event. The earthquake triggered a transoceanic tsunami, recorded by all the tide gauge stations in the Pacific Ocean. In Hawaii, they registered a 9-m wave; on the Chilean coast, 16,000 km away from Kamchatka, waves rose almost 2 m high (in the southern part of Chile, it became the strongest tsunami event from 1920 to 1960, i. e., in almost 40 years).
The Kamchatka tsunami of 1952 became the first event that required the involvement of the International Tsunami Warning Service in the Pacific Ocean, established in the early 1950s, with a center in Honolulu (Hawaii). Early-time warnings about hazardous waves helped avoid casualties outside the nearest zone, directly adjacent to the earthquake source. Back then, the Soviet Union was not a member of that system, but in any case, a warning received from Honolulu an hour after the earthquake (in those days, communication technology and data processing could not work faster) would have been too late.
However, conclusions were made from the past catastrophe. The USSR Academy of Sciences initiated research into the tsunami problem; the first scientific publications appeared. In 1957, the USSR Government took a decision to establish a Tsunami Warning Service (TWS) in the Far East. The Academy of Sciences was entrusted with developing its work principles, and several design bureaus, with special equipment for the TWS. The next year (back then, government decisions were implemented very fast), this service started working and made its first forecast during the Urup earthquake on November 7, 1958, which triggered a dangerous tsunami in the South Kuril Islands.
One megatsunami equals a dozen ordinary tsunamis, also hazardous and destructive
Historical catalogues identify a transoceanic tsunami by a formal criterion, i. e., splash heights of more than 5 m at a distance of more than 5000 km from the tsunami source. In physical terms, this criterion means that the tsunami is able to perpetrate destruction on the opposite shores of the ocean basin. It turns out that among more than 2250 tsunamigenic events that have occurred in the World Ocean from the 2nd millennium BC to the present time, there have been only 18 such events, i. e., less than 1 % of the total. However, these events are responsible for more than a half of the total number of tsunami fatalities and for a substantial proportion of material damage.
The remaining 99 % are local and regional tsunamis. The splash heights they create on the near coast may be substantial, reaching tens or even hundreds of meters (with a record height of 525 m in Lituya Bay, Alaska, during a coastal landslide caused by the 8.0 magnitude earthquake on July 10, 1958). However, the tsunami hazard zones for these events are small in size, ranging from several hundred meters (in the case of local tsunamis caused by landslides) to the first hundreds of kilometers (in the case of strong tsunamis of a seismotectonic origin).
In contrast, the hazardous effects of major transoceanic tsunamis can manifest themselves on much larger areas of the coast, extending 700—800 km or even more. The most important thing, however, is that these tsunamis can perpetrate destruction in the far zone (more than 1000 km from the source), as far as the opposite shores of the ocean, which never happens during normal, even very destructive regional tsunamis. The latter may cause large flood heights of 20—30 m; however, the splash zone is limited to the coastal segment closest to the source, beyond which wave heights diminish rapidly. In the far zone, these tsunamis can be detected only instrumentally.
If we analyze a list of the currently known transoceanic tsunamis with their main parameters (Gusyakov, 2014), we can see that 15 of the 18 transoceanic tsunamis arose from earthquakes of magnitude 9.0 or more. This is true, at least, of all the events since the mid‑20th century, when it became possible to instrumentally measure magnitudes in the long-period region.
Based on the data in this list, we can take a magnitude of Mw = 9.0 as a threshold for distinguishing transoceanic megatsunamis from all other tsunamigenic events. It appears that even a slight decrease in Mw (by 0.2—0.3), given the same destructive potential of the event in the near zone, leads to a sharp decrease in the hazard for remote coasts. The last such example was the Maule tsunami in Chile on February 27, 2010 (Mw = 8.8), whose maximum splashes on the Chilean coast reached 25—29 m. However, the wave heights in Hawaii, in Japan, and on the Kuril–Kamchatka coast, did not exceed 1—2 m. Although sounding an alarm on these coasts was fully justified, this wave posed no real threat and caused no damage in Hawaii or Japan.
Another important feature of transoceanic megatsunamis is their ability to penetrate into marginal seas and exert a dangerous impact on coastal areas without subduction zones (i. e., about 80 % of the coast of the World Ocean). It is known that most tsunamigenic earthquakes occur precisely in the subduction zones. Strong (up to 8.5) earthquakes arising here, in these zones may induce hazardous tsunamis with heights of 15—20 m on the nearest islands, but their impact zone is limited by the source size to 200—300 km. A typical example is the last destructive tsunami in the Middle Kuriles on November 15, 2006 following an 8.3 earthquake. On Simushir Island, the splash heights reached 20—22 m, while on the South and North Kuriles, they did not exceed 1 m, and on the Okhotsk coast of Kolyma, the waves had amplitude of no more than 30 cm.
However, megaquake-induced tsunamis, with completely different characteristics, can pass through chains of islands and cause substantial disturbances in the waters of marginal seas. This important result, first discovered by the Novosibirsk Tsunami Research Group (Beizel et al., 2014), is confirmed by observational data from real historical events. In Magadan, for instance, the greatest sea level fluctuations with amplitude of over 2 m were observed during the 1952 Kamchatka tsunami and the 1960 Chilean tsunami. In the Koryak village of Pakhachi, the amplitude of level fluctuations reached 7 m in 1960, a record value for this part of the Bering Sea. As for other countries, the coastline of the Pacific island systems such as Hawaii, Tahiti, Tonga, and the Marquis Islands suffer mainly from distant tsunamis generated by M9 class subduction earthquakes occurring in various parts of the Pacific Ring of Fire.
Where will it happen next?
Most of the identified megatsunamis (16 out of 18) occurred in the Pacific Ocean; one in the Indian Ocean (2004, Indonesia), and one in the Atlantic (1755, Lisbon). Importantly, this list of megaevents appeared only in recent years, when after the two devastating tsunamis in the beginning of this century (the Indonesian tsunami of 2004 and the Tohoku tsunami of 2011), scientists set out to closely examine the data on strong tsunamis in the past. Previously, we knew only about five transoceanic tsunamis (Aleuts, 1946; Kamchatka, 1952; Aleuts, 1957; Chile, 1960; Alaska, 1964). Earlier events appeared on this list after revising the parameters of several past tsunamis based on new evidence about their spatial scale and the results obtained by studying their geological deposits.
A prime example comes from the works of US geologists and geomorphologists from the University of Washington, who attempted to reconstruct the history of major earthquakes in Cascadia, the northwestern United States. Compared with the neighboring California, this region has a much lower level of modern seismicity. All the more surprising was the discovery of obvious geological traces of major earthquakes and ensuing tsunamis that occurred here in prehistoric times. The last of these megaquakes occurred in the Seattle area about 300 years ago, before the arrival of the first Europeans. Its echoes (“a strong earthquake that happened on a winter night”) on the eve of the 20th century remained in the legends of local Indians. The radiocarbon dates of the tsunami deposits fell within a range of 1700 ± 20, and the dendrochronological dating of the trees that perished when the coastline submerged below the tidal level narrowed this interval to 1700 ± 2. The devastating tsunami hit at least 1000 km of the coastline in Cascadia and must have surely spread across the Pacific Ocean. Indeed, targeted searches in Japanese archives revealed reports of unusual fluctuations in sea level, unpreceded by an earthquake, across Japan’s east coast on the night of January 27—28, 1700. Now this paleoevent has been included into the catalogs of tsunamis and earthquakes with sufficiently reliable parameters: the source coordinates (39.5° N, 142.0° W), the magnitude (9.0), the date (January 27, 1700), and even an estimate for the time (6 a. m. GMT). The outcome of this unique, truly interdisciplinary research has been a series of publications in prestigious scientific journals, including Science and Nature, and a monograph The Orphan Tsunami of 1700 (Atwater et al., 2005).
Until the beginning of this century, scientists believed that megaquakes capable of generating transoceanic tsunamis could occur only in some special areas of subduction zones. As regards the mechanism underlying these megaevents, they put forth several hypotheses. Initially, they thought, if the oceanic plate is relatively young, i. e., hot and light, then it should undergo subduction at a smaller angle, thereby providing greater adhesion to the overlying continental crust and allowing the accumulation of large stresses, which are released during extremely strong events. While analyzing the four megaquakes known at that time (Kamchatka, 1952; Aleuts, 1957; Chile, 1960; Alaska, 1964), the US seismologists Larry J. Raff and Hiroo Kanamori found that all of them occurred in subduction zones characterized by ages less than 80 Myr and subduction rates of more than 5 cm per year (Ruff and Kanamori, 1980). This finding rested on some physical foundations, such as that the less dense oceanic crust requires greater effort to sink below the continental crust. However, the presence of a tangential component in the relative motion of the plates and the occurrence of a thick layer of sediments in the deep-water depression were considered as factors reducing the likelihood of megaquakes. From this point of view, the 2004 magnitude 9.0 earthquake near Sumatra came as a bombshell for seismologists. Previously, this part of the subduction zone was considered capable of generating only magnitude 8.0 earthquakes. Even more astonishing was the fact that given the overall tangential motion of the plates, the predominant mechanism underlying the main shock was a low-angle underthrust, with the shift along the fault line being almost transverse to the contact zone of the plates.
The 2011 magnitude 9.0 earthquake in the most tsunami-active region of Japan (Tohoku), where no such earthquakes had been recorded in historical time, ultimately convinced most seismologists that megaquakes can occur anywhere in subduction zones. The only reason why some zones present no evidence of such events comes from the insufficient length of the instrumental and historical catalogs, which in most regions cover a much shorter timespan than the recurrence period of megaquakes.
The Commander breach: A threat to the Far East coast?
A map of the sources of tsunamigenic earthquakes shows a salient breach near the Commander Islands, with no evidence of strong earthquakes throughout historical time. This breach was first mentioned almost half a century ago by Russian and foreign seismologists. Now its seismic potential has aggravated since GPS observations detect a continuous relative displacement of the Bering Plate relative to the Pacific Ocean at a rate of about 30—50 mm per year. No doubt that sooner or later, this potential will discharge itself as a strong earthquake; the only question is its magnitude and the specific mechanism at its source. The “seismically quiet spot” extends to about 500—600 km, enough to accommodate a magnitude 9.0 earthquake capable of generating a transoceanic tsunami. The answer to the question of whether it poses a serious threat to the coast of Kamchatka and the Kuril Islands largely depends on the mechanism at the source of the future megaquake. Determinations of mechanisms underlying the weaker earthquakes that occur from time to time in this zone show a significant role of the shear component, which reflects the predominantly lateral displacement of the plates relative to one another. Sources of this kind excite much weaker (by an order of magnitude) tsunamis than those with a predominantly upthrow- or upthrust-based shift. However, as noted above, a similar situation, characterized by an oblique displacement of plates, occurred in the Sumatra–Andaman zone, whereby the shift along the fault line of the 2004 earthquake proved to be almost upthrust-based and oriented transverse to the arc.
The worst-case scenario for the future Big Commander Earthquake, considered by Russian seismologists and geotectonists (Lobkovsky et al., 2014), yields 8—10-m tsunami waves for the entire east coast of Kamchatka, which will undoubtedly destroy the coastal infrastructure retained here since the Soviet times. No tsunamis of this strength have been observed here since 1952, and to the north of the Kronotsky Peninsula, since 1923, i. e., for almost a century. The waves will also affect the Kuril Islands, most likely without causing a big catastrophe: tsunami waves cannot propagate over long distances along the coastline because the wave energy constantly “swerves” to the shore due to refraction on the sloping bottom (continental slope and shelf). The earthquake and tsunami pose the main danger for the population of the Commander Islands although the largest settlement there (the village of Nikolskoe), located on a high (up to 20 meters) coastal terrace, is well protected from tsunamis.
When will it happen next?
Earthquakes of the highest possible magnitude (9.0—9.5) occur very rarely, but they cause the greatest damage and loss of human lives.
The displacement along the fault line at the sources of these earthquakes may reach 25—50 m or more. The relative rate of movement of the plates in the subduction zones varies from 25 to 100 mm per year. Then, if we assume that all the accumulated stresses are released only at the sources of major earthquakes, their recurrence period should lie in the range from 1000 to 250 years. If some of the deformations are released as aseismic creep also at the sources of smaller earthquakes, then this period may be even longer. The actual timespan between two successive megaevents varies widely: for the known historical earthquakes, from 215 years (Kamchatka, 1737 and 1952) to 1142 years (Tohoku, 869 and 2011). The longest series of observations of such events, which extends to the present time from almost 10,000 years ago, exists for the Cascadia subduction zone in the northwestern United States. The chronology of events here was reconstructed from the data acquired by drilling the adjacent areas of the seabed, where sedimentary columns contain distinct traces of turbidite flows initiated by strong earthquakes. It turns out that the average recurrence period of major earthquakes in this zone is about 650 years, but the actual timespan between two successive events varies from 300 to 1500 years.
Estimating the actual period of recurrence for these events poses the main challenge for their long-term forecasting. Since they happen very rarely, for most regions not scrutinized by geologists, unlike in the northwestern United States, the recurrence of megaevents defies direct calculation, necessitating estimates by indirect methods, e. g., by linear extrapolation to large magnitudes (8—9) of the recurrence pattern for moderate earthquakes (magnitude 5—7), reliably described by the Gutenberg–Richter law. However, in some regions the actual recurrence of extremely strong earthquakes significantly (by a factor of 3 to 5) exceeds the estimate derived from the Gutenberg–Richter law.
Another way to estimate the recurrence of megaevents is to search for and analyze the geological traces of tsunamis on the coast. These methods are now well developed and widely applied in various parts of the oceanic coast, including on the coast of Kamchatka, the Kuril Islands, and Primorye. Over the many years of field research, the Institute of Volcanology and Seismology, Far East Branch, Russian Academy of Sciences (FEB RAS), and the Pacific Institute of Geography, FEB RAS, revealed dozens of new, previously unknown events in this region over the last 3000—5000 years, largely expanding the tsunami catalogues for the Kuril–Kamchatka coast. Still, a big issue remains: How to identify traces of actual megatsunamis among these events? The standard criterion, i. e., the splash height and distance, does not work here because unusual earthquakes of magnitude 7.0—7.5 may sometimes occur in the subduction zones, generating tsunamis that affect a small portion of the coast yet give local splashes of 15—20 m (the so-called tsunami earthquakes, a term introduced by Kanamori). The only way to single out megatsunami deposits among other similar events is through careful control along the coastal stretch of the maximum splash zone. This requires, however, a lot more field work, which poses a great challenge under the severe conditions of Kamchatka and the Kuril Islands.
To date, only one work has been published (Razzhigaeva et al., 2017) with an attempt to summarize the results of the numerous finds of paleotsunami traces on the South Kuril Islands to identify possible megatsunamis. The authors analyze the spatial scale of paleotsunami traces on the South Kuriles over the past 2500 years to identify the strongest events that can be considered as megatsunami candidates. Approximate dating for these events places them in the 17th, 13th, and 10th centuries and in the intervals 1400—1600, 1700—1800, and 2000—2100 years ago. On top of finding the spatial scale of paleoevents, another challenge comes from their dating, which, as a rule, requires expensive radiocarbon analyzes. However, putting this issue in the context of long-term tsunami hazard assessment makes it simpler. Since these estimates are largely probabilistic, one does not need to know the exact dates of the events; their total number over a certain period would suffice. These estimates can be obtained even in the field conditions, using a tefrochronological scale, well developed for Kamchatka and the Kuril Islands.
Protecting from 20-meter waves
The Tohoku coast, most affected by the megatsunami on March 11, 2011, is morphologically a ria coast, a steep, mountainous coastline indented by narrow inlets adjoining the valleys of rivers flowing into the ocean. In fact, these valleys are the only place suitable for residential houses over the more-than‑900-km coastline. Now almost all of them are blocked by protective dams 7—8 m high, which effectively protect the residential areas behind them. Still, these structures failed to protect the people from the 12—15-m wave that hit this coast on March 11, 2011. However, it is not only extremely expensive to build higher protective dams of 15—20 m—it is often utterly impossible to allocate land plots in densely built-up areas to lay the foundations of these massive structures.
One of the implications of the 2011 tsunami in Japan was the adoption of the so-called two-tier tsunami protection strategy. The government officially admitted that the existing coastal defenses could protect the population from ordinary tsunamis whose heights do not exceed 5—7 m but prove useless against the rare megatsunamis with heights of 15—20 m. Therefore, all critical facilities (schools; hospitals; educational, shopping, and cultural centers) should be gradually removed from the hazard zone. In the overpopulated Japan, where all coastal land plots suitable for construction have long been occupied, it is virtually impossible to find new sites for these buildings. Therefore, the strategy includes engineering works to transform the coastal relief in order to artificially create flat platforms for these objects. However, such a practice of planning and construction in tsunami-hazardous zones might work effectively in Japan but hardly worldwide due to its high cost.
The ultimate goal of studying natural disasters is to develop strategies and practical recommendations for decision-makers on how to optimize the potential risks and costs of preventive and protective measures. An optimal response seeks a balance between the expected losses from a natural disaster and the economic costs of preventing them (Stein and Stein, 2012). Achieving this balance is not an easy task because the decision-makers are themselves not experts in this field and tend to treat scientists’ recommendations as an ultimate truth. However, the experts and scientists who give these recommendations have a limited ability to predict future events; therefore, they must inform the decision-makers, honestly and frankly, about all the uncertainties associated with the risk estimates.
Any expert forecast should be as transparent as possible, which means that the decision-makers should be made aware of the underlying assumptions, hypotheses, process models, data, and uncertainties. It is absolutely necessary to clearly indicate the level of uncertainty even if it cannot be strictly calculated. Unfortunately, this is not always the case. The actual uncertainties for many of the published seismic and tsunami hazard maps prove to be much larger than those indicated by the map compilers, which often derive them from computation techniques only.
Литература
Atwater B. F., Musumi-Rokkaku S., Satake K. et al. The orphan tsunami of 1700; Japanese clues to a parent earthquake in North America: U.S. Geological Survey Professional Paper 1707. Seattle, 2005. 133 p.
Beizel S. A., Gusiakov V. K., Chubarov L. B., and Shokin Yu. I. Assessing the impact of distant tsunamis of the Far East coast of Russia from mathematical simulations // Izv. Ross. Akad. Nauk. Fiz. Atm. Okean. 2014. V. 90. N. 5. P. 578–590 [in Russian].
Gusiakov V. K. Residual displacements on the surface of an elastic semispace // Uslovno-korrektnye zadachi matematicheskoi fiziki v interpretatsii geofizicheskikh nablyudenii (Conditionally Correct Problems of Mathematical Physics in the Interpretation of Geophysical Observations), Novosibirsk, 1978, P. 23–51 [in Russian].
Gusiakov V. K. Strongest tsunamis in the oceans and coastal safety hazard // Izv. Ross. Akad. Nauk. Fiz. Atm. Okean. 2014. V. 90. N. 5. P. 496–507 [in Russian].
Lobkovsky L. I., Baranov B. V., Dozorova K. A., et al. Commander seismic breach: earthquake forecast and tsunami calculation // Okeanologiya. 2014. V. 54. N. 4. P. 561–573 [in Russian].
Razzhigaeva N. G., Ganzei L. A., Grebennikova T. A., et al. Problem of megatsunami paleoreconstructions in South Kuriles // Tikhooken. Geol. 2017. V. 36. N. 1. P. 37–49 [in Russian].
Ruff L., Kanamori H. Seismicity and the subduction process // Physics of the Earth and Planetary Interiors 23. 1980. Issue 3. P. 240–252.
Stein J., Stein S. How good do natural hazard assessments need to be? // GSA Today 23. 2012. № 4/5. P. 60–61.
Titov V. V., Kânoğlu U., Synolakis C. Development of MOST for real-time tsunami forecasting // J. Waterw. Port Coast. Ocean Eng. 2016. V. 142. N. 6. 03116004, doi: 10.1061/(ASCE)WW.1943-5460.0000357.
