
Nucleic Pilgrims
What are free (extracellular) nucleic acids? They are a source of nitrogen and phosphorus for bacteria living in soil and in sea deposits, and an alarm signal for the cell infected with a pathogen. However, recent decades have demonstrated that the role of nucleic “pilgrims” extends further. Each year brings ever new data on these molecules, which in many respects determine the operation of biological systems, from an individual cell to a population
What are free (extracellular) nucleic acids? They are a source of nitrogen and phosphorus for bacteria living in soil and in sea deposits, and an alarm signal for the cell infected with a pathogen. However, recent decades have demonstrated that the role of nucleic “pilgrims” extends further. Each year brings ever new data on these molecules, which in many respects determine the operation of biological systems, from an individual cell to a population
Nucleic acids, DNA and RNA, carry the information about the structure, development, and reproduction of all cells in a living organism. Thus, it is no wonder that the cells carefully guard these structures from potentially dangerous external factors. In particular, the genomic DNA of bacteria is compactly packed with the help of specialized proteins and protected from the environment by a stable cell wall. As for higher organisms, their DNA is stored in the cell nucleus within complex structures, chromosomes.
It has been long believed that nucleic acids leave the cell only after its death. Indeed, it is known that bacterial DNA is transferred from one cell to another during the reproduction of bacteria; however, even in this case DNA does not migrate freely but is confined to a specialized protein complex. Even the DNAs and RNAs of “relatively live” viruses, a sort of autonomous genetic program, travel in extracellular space in a container of specialized transport structures.
However, recent studies have demonstrated that “free” (extracellular) nucleic acids are abundant not only in naturefor example, in soil, but also in living organisms themselves. What are the role and specific functions of these “freewheeling” nucleic acids?
A genetic “intervention”?
Nucleic acids are rather stable polymeric molecules and thus are able to persist in natural surroundings for a long time after the death of the organism. For example, relatively long DNA fragments remain almost unchanged in the bone tissue for several tens of thousands of years (!), which allows for paleogenetic studies, such as decoding the genome of a mammoth or a Neanderthal.
The concentration of bacterial DNA in the upper 10-cm layer of ocean bottom sediments is about 0.5 g/m2, and over 90% of this DNA is “free” (Dell’Anno and Danovaro, 2005). As for the total amount of DNA falling onto the ocean bottom annually, it exceeds 12 billion tons! Evidently, such an amount of potential nitrogen and phosphorus sources has a significant effect on the life activities of marine microorganisms.
As for the soil, where the DNA of plant and bacterial origins can persist for months and even years, its concentration reaches 2 µg/g (Niemeyer and Gessler, 2002). Interest in such DNA is currently fuelled by the subject of genetically modified organisms (GMO), since their recombinant DNA after their death finds itself in soil. There were some concerns that such “modified” genes would be assimilated by soil bacteria and then passed to other organisms via the so-called horizontal gene transfer, a sort of “parallel” evolution characteristic of microorganisms. So far, there is no direct evidence for any transfer of genetic information from genetically modified plants to bacteria; moreover, the very probability of such a process under natural conditions is extremely low.
However, horizontal DNA transfer sometimes engenders problems. This refers to bacterial films (the so-called biofilms) formed on solid surfaces, including the surfaces of prosthetic implants, teeth (causing dental caries), and on bronchial walls in the case of bronchitis and pneumonia.
Such films consist of reproducing bacteria connected with one another and with the surface by a polymeric hydrated substance, a mixture of polysaccharides, proteins, DNA, and RNA (Nishimura et al., 2003). Note that nucleic acids appear in the intercellular substance of biofilms not only from dead bacterial cells, but also as a result of a specialized secretory process, which is stimulated by “cocultivation” of different bacterial species (Hamilton et al., 2005). These DNAs are readily conveyed from one bacterium to another, making biofilms a big problem for therapists: the pathogens contained there not only escape the adverse factors, but also successfully evolve via genetic exchange into resistant strains (in particular, antibiotic-resistant).
As for GMO consumption by humans, note that every day considerable amounts of alien DNAs of manifold origins enter our body with food. It is known that people who eat genetically modified soy beans, the most widespread transgenic product, successfully digest the specific transgene in their gastrointestinal tract (Netherwood et al., 2004).
However, this is not always the case with the “eaten genes”. Experiments with laboratory animals have shown that DNA fragments of digested food two days after its consumption may enter the blood and linger in liver and spleen cells (Hohlweg et al., 2001). However, animal cells neither transcribe the corresponding RNAs nor synthesize the proteins encoded by plant genes. This is also true for transgenic DNAs, as has been confirmed by the experiments when laboratory mice were fed GMO products for eight generations (Hohlweg et al., 2001).
Thus, no direct danger associated with consumption of transgenic prodfrom of foreign DNA, so abundant in the environment, would had to emerge during the evolution.
Nonetheless, the relationship between us and our food is not so simple. According to recent studies, RNAs of a plant origin can retain their biological activity in the animal body! In particular, Chinese researchers have succeeded in demonstrating that cells of the gastrointestinal tract and blood of laboratory mice and humans contain some microRNAs from their food (rice and cruciferous plants); moreover, the microRNA concentrations are rather high. This foreign microRNA is able to inhibit the expression of one of the genes that encode a low density lipoprotein receptor (Zhang et al., 2012).
Blocking aggressors
Not only do living cells carefully protect their genetic programs, but they also actively struggle with the foreign ones. Once the host cell is infected with a virus or a bacterium, the host’s first response consists in recognizing and destroying foreign nucleic acids; or the infected cell switches on the mechanisms of suicide to prevent the infectious agents from spreading in the body.
The system of innate nonspecific immunity, which responds to foreign molecular substances, emerged at an early stage of the evolution of multicellular organisms. This system is able to recognize a “foreigner” due to the presence of certain conserved nucleotide sequences in its RNA or DNA. For the bacterial DNAs, such recognizable sequences are the regions containing hexameric clusters of cytosine–guanine dinucleotides (CpG). Unlike mammalian DNAs, these sequences in bacteria are nonmethylated.
When phagocytizing immune cells (macrophages, neutrophils, eosinophils, and others) destroy the bacterial envelope, the nonmethylated CpG sequences become “visible” to the toll-like receptors (TLRs) of phagocytes, which are able to recognize foreign nucleic acids. Activation of the intracellular receptor TLR9 triggers a cascade of molecular events that determine the subsequent development of immune response. B and T lymphocytes are activated and start to intensively produce specialized substances, cytokines, which coordinate the development of the inflammatory response; in addition, immunoglobulin production is increased (Hacker et al., 2002). Finally, the antibodies specific to the corresponding pathogen are produced.
In the case of a viral infection, the alarm is raised by the presence of a double-stranded RNA, characteristic of viruses (unlike DNA, the RNA “common” for the cell is formed of a single nucleotide strand). Such a double-stranded RNA is either present within virus particles or appears in the infected cell during virus reproduction.
The recognition of double-stranded RNA as an alert is mediated by a phagocyte surface receptor, TRL3, which triggers the signaling cascade that activates the intensified synthesis of antiinflammatory proteins, interferons (Yu et al., 2011). Interferons specifically bind to cell receptors and activate certain genes responsible for the antiviral response. An example is the gene encoding the enzyme RNA-dependent protein kinase (PKR), which induces apoptosis (cell suicide) or the protein complex OAS/RNAseL, which inhibits replication and translation of the virus RNA.
Eventually, the cell infected with a virus usually loses its ability to divide and synthesize proteins and is frequently eliminated from the body.
Another typical response of an infected cell to activation by the presence of a viral double-stranded RNA is RNA interference, an avalanche-like process leading to cleavage of foreign RNA and switch-off of viral genes.
Self comes first
All the above mentioned refers to the so-called exogenous nucleic acids, which are foreign to the body. On the other hand, a long time ago it was found that animal and human blood, contain endogenous, i.e. their own, extracellular DNAs and RNAs. However, the presence of endogenous (own) extracellular DNAs and RNAs in the animal and human blood was also demonstrated rather long ago.
In higher plants, extracellular RNAs can migrate between neighboring cells and be transported to remote organs and tissues via the phloem, a conductive vascular tissue, which provides a descending transport of organic substances (photosynthetic products) from leaves to other organs. This has been demonstrated in the experiments on transplantation of plant parts. In addition, a specialized phloem protein was discovered; it binds single-stranded RNAs and enhances their transfer both between adjacent cells and through the filtering structures in the phloem.
Messenger RNAs of plant genes, microRNAs, small interfering RNAs, as well as several classes of small RNAs with yet unknown functions have been detected in the circulating flow of the phloem; in addition, this flow contains a large amount of exogenous RNA: pathogens, for example, viruses can use this route for spreading their genetic information (Lough and Lucas, 2012).
In mammals, the extracellular DNAs are population of molecules with a length of 180 to 3500 base pairs. One of the sources for these molecules is the cells destroyed by apoptosis or necrosis (Jahr et al., 2001). This pattern is characteristic of tumor cells as well as of the cells injured by traumas, myocardial infarction, severe inflammation, and even by vigorous physical exertion.
INTERCELLULAR “MAIL”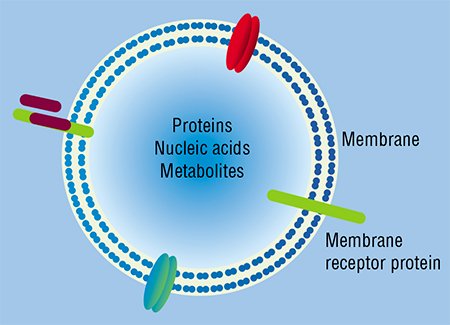
It is well worth noting that F. Miescher, a Swiss chemist, isolated from cell nuclei an earlier unknown substance and named it “nuclein” in the same year of 1868. Miescher’s works laid the foundation for research into nucleic acids – DNA and RNA.
As is not uncommon in the history of science, both the concept of intercellular transfer of genetic information and the mechanistic model of gemmule as its carrier have appeared in demand in state-of-the-art biology, matching the classical molecular genetic “background”, which regards nucleic acids as the depositary and carriers of genetic information.
However, the “interpenetration” of ideas did not start at once. On the one hand, the micro- and nanoparticles secreted by animal cells and able to “travel” in the body were discovered. Unfortunately, these studies almost did not join with the research into extracellular nucleic acids discovered in blood and in other biological fluids. In particular, the granules released by platelets were interesting to researchers mainly because of their proteins and lipids that were directly involved in clotting, while an amazing stability of RNA in blood plasma and serum was explained by the fact that it circulated within the fragments of destroyed cells.
Everything changed when it was found that membrane particles – microvesicles and exosomes released by some cells and ingested by others – contained RNA (Valadi et al., 2007). This clearly demonstrated the ability of viable cells to actively exchange nucleic acids.
According to current concepts, the majority of cells in the body of a human and higher animal are able to secrete membrane particles with a size of 50 to 1000 nm. Once separated from a donor cell, these particles may be ingested by either neighboring or distant recipient cells. The donor cell will recognize the intercellular “message” sent to it according to the specific features in protein and lipid composition of the particle membrane external surface, which are a kind of “shipper’s seal” and “recipient’s address”.
Currently, research into secretion and circulation of cellular macro- and nanoparticles is among the most booming areas in biology, the most intriguing issue being the informational content of intercellular messages and its utilization in recipient cells.
First and foremost, note manifold types of RNA present in the contents of exosomes/microvesicles, ranging from messenger RNA coding for proteins to noncoding microRNAs. Although some data suggest that protein may be synthesized on such mRNA in recipient cells (Valadi et al., 2007), many details of this process (in particular, efficiency of protein synthesis on the RNA “foreign” to the recipient cell and most likely fragmented) raise reasonable doubts of theoreticians and experimenters.
On the other hand, the noncoding RNAs (including microRNA) in the cells, directly or indirectly, are regulators of nearly all key vital cellular processes (moreover, even partially hydrolyzed noncoding RNAs retain this ability). Thus, it is hardly surprising that the set of noncoding RNAs is now regarded as the main informational content of all the circulating membrane particles.

Research into RNAs circulating in human blood plasma began before it was discovered that they circulated within exosomes and microvesicles (Kolodny and Culp, 1972; Stroun et al., 1978; Wieczorek et al., 1985).
The Institute of Chemical Biology and Fundamental Medicine, Siberian Branch, Russian Academy of Sciences (Novosibirsk, Russia) started research into circulating nucleic acids in the early 2000s. Free DNAs and RNAs were soon detected not only in blood plasma, but also on the surface of human cells, which deepened the insight into nucleic acid circulation in the body and confirmed their postulated interaction with cells. It was also demonstrated that DNAs and RNAs of blood are very helpful in diagnosing rumors; their analysis results not only in most precise diagnoses, but also in distinguishing between malignant and benign neoplasms (Rykova et al., 2004, 2008; Skvortsova et al., 2006).
State-of-the-art techniques, including massively parallel sequencing, are now used at the Institute of Chemical Biology and Fundamental Medicine to obtain a comprehensive description of all RNA types present in human blood. This makes it possible to detect hundreds of millions of nucleotide sequences in a single experiment, to find, among them, tens of thousands of known sequences, and to discover new, so far undescribed sequences.
The Laboratory of Biotechnology with this Institute is involved in both decoding of the informational content of the RNAs circulating within exosomes and microvesicles, and in the search for new kinds of regulatory RNAs, including those appropriate as markers for cancer diseases.
A set of RNA species circulating in the blood of healthy individuals was successfully characterized using a SOLiD platform for highly parallel sequencing (Semenov et al., 2012). The RNAs circulating in the blood of non-small-cell lung carcinoma patients were analyzed in collaboration with the medical specialists of the Novosibirsk Regional Cancer Center. As a result, several new RNA markers for human cancer diseases were discovered. This set of markers includes messenger RNA fragments, fragments of various noncoding RNAs, as well as new transcripts not described earlier.
Unique data confirming a considerable contribution of microvesicles and exosomes to the pool of extracellular RNAs circulating in the human blood were also obtained.
In order to improve and expand the methodology for isolation and analysis of extracellular micro- and nanoparticles, the Institute of Chemical Biology and Fundamental Medicine together with two other institutes of the Siberian Branch of the Russian Academy of Sciences – Institutes of Inorganic Chemistry and Semiconductor Physics – initiated, in 2012, an integrated interdisciplinary basic research project to solve both theoretical problems associated with exosome and microvesicle functions and applied tasks that involve new approaches to diagnostics and treatment of human diseases with the help of circulating membrane complexes.
Siberian Branch, Russian Academy of Sciences, Novosibirsk)
However, extracellular DNAs and RNAs are also actively secreted by fairly live, fully functional cells. In particular, extracellular DNA of a tumor origin appears in the blood at early stages of carcinogenesis, when necrosis and apoptosis are almost unobservable in the tumor. It is known that some cultivated human cells (for example, endotheliocytes of the umbilical vein or peripheral lymphocytes) secrete DNA into the incubation medium (Morozkin et al., 2004). In addition, phagocytes are also involved in emergence of free nucleic acids by ingesting products of cell destruction and repeatedly secreting them in a “digested” state.
Concentration of extracellular DNAs and RNAs is considerably influenced by specialized cleavage enzymes, which are abundant in the blood; DNA is saved only because outside the cells it appears either in complexes with proteins, for example, with histones as nucleosomes, or packaged into membrane structures, such as exosomes, microparticles, or apoptotic bodies (Stroun, 2000).
Interfering RNAs of some primitive animals, such as the parasitic nematode C. elegans, spread themselves throughout the whole body with the help of a specialized protein, SID-1 (Feinberg et al., 2003). In mammals, such RNAs are transported in complexes with the proteins involved in RNA interference or with high density lipoproteins, a “good” cholesterol (Vickers et al., 2011). When entering the blood, the complexes containing nucleic acids can bind to proteins of the blood plasma or cells (erythrocytes and leukocytes).
All these structures are ingested by both neighboring cells and by those located at a considerable distance from the secreting “parental” cell. The mechanisms underlying penetration of nucleic acids into cells are still vague since the phospholipid cell membranes are a barrier for their passive diffusion. The interaction between the “vehicles” – vesicles and protein complexes – and surface proteins of the cell plays a key part in this process. However, some data suggest that recognition and ingestion of a “nude” nucleic acid may also take place in some cases.
To the benefit…
Extracellular nucleic acids synthesized in the body and involved in general circulation (this especially refers to RNA) play the most important signaling role in both the local and remote regulations of organ and tissue development by coordinating cell functioning in multicellular organisms.
Thus, experiments aimed at finding the factors that control cell differentiation and tissue morphogenesis helped to discover the RNA–protein complexes named angiotropins. The RNA of these complexes is represented by highly modified short (with a length to 200 nucleotides) sequences. These molecules interact with proteins in the presence of metal ions (Cu, Ca, Na, or K) to form complexes acting as antiinflammatory factors (cytokines) and as regulators of differentiation for the cells that line capillaries when these structures are formed (Wissler, 2004).
In the last few years, a lot of attention has been focused on microRNAs, short (19—24 nucleotides) single- or double-stranded (“immature”) molecules involved in expression inhibition of cell genes via RNA interference. Only a short time ago, this specific regulation of protein synthesis was regarded as a local event of an intracellular scale. However, it has been recently shown that high concentrations of active, interfering microRNAs are present in the general circulation despite high activitys of extracellular RNA-cleaving enzymes.
The microRNA transfer between cells may enhance development of most diverse physiological effects from regulation of the immune response to cell migration. In a pregnant woman, the microRNAs secreted by embryonic tissues (this RNA is actually foreign to the mother’s body) may be involved in adapting the maternal organism to pregnancy (Mincheva-Nilsson et al., 2010), and the microRNAs of the mother’s milk, in turn, in developing the baby’s immune system (Kosaka et al., 2010).
The messenger and microRNAs transported in exosomes may protect cells from apoptosis and stimulate their division. It is this fact that explains the beneficial effects of stem cell injections into injured organs. Earlier it was believed that stem cells give rise to new tissue in injured organs, whereas the point is that they produce exosomes, which help the cells of the injured tissue to survive and reproduce (Biancone et al., 2012).
It has been also experimentally demonstrated that a “cocktail” of mRNAs and/or microRNAs may be used to obtain an opposite effect: they allow for induction of stem cells from already differentiated ones, for example, fibroblasts (Jayawardena et al., 2012).
... and to the detriment of the organism
In particular, tumor cells (for example, of glioblastoma) actively secrete exosomes with microRNAs, which influence the cells of blood vessels and enhance metastasizing (Skog et al., 2008). Incidentally, exogenous microRNAs can act in a similar manner: the genome of Epstein-Barr virus encodes the microRNAs that penetrate neighboring cells when secreted by an infected cell within exosomes and interfere with the expression of a cytokine that is responsible for activating the cell immune response (Pegtel et al., 2010).
This is not the only example of a negative impact of extracellular nucleic acids. Thus, in several instances extracellular DNA is directly involved in developing pathologies. The best known disease of this type is systemic lupus erythematosus. In this autoimmune disease, the body accumulates antibodies pathogenic to its own DNA (Rumore et al., 1990). These antibodies form complexes with extracellular DNAs which enhance inflammatory responses. The disease is believed to be triggered by disturbances in the mechanisms that regulate the level of circulating DNAs, since many patients display decreased activity of both the enzyme cleaving DNA and phagocytes utilizing it (Napirei et al., 2006).
Since cells are able to ingest cell degradation products, a malignant transformation of cells induced by gene transfer from tumor cells killed by apoptosis was postulated. In the case of such a mechanism, cancer could be transmitted as an infectious disease, via blood, from cell to cell of an affected organism, forming remote and close metastases.
According to this hypothesis of “genometastases”, cancer spreads in the body as a result of DNA circulation (or apoptotic bodies) rather than via tumor cells (Garcia-Olmo et al., 2012). Although this hypothesis has not been distinctly proved in experiments, there are no grounds to reject it.
All currently available data unambiguously demonstrate the paramount biological significance of extracellular nucleic acids. According to the boldest assumption, free nucleic acids are not mere signaling molecules but rather an important component of the mechanisms acting both at the level of an organism and population, and eventually at the evolutionary level.
Genometastases, horizontal gene transfer, reprogramming of neighboring cells, and even transfer of the extracellular “genome” to future generations, which bypasses the neo-Darwinian mechanisms of inheritance – all these phenomena, fantastical at first glance, will be undoubtedly studied in detail in the nearest decade along with the already traditional research into the sources, causes, and mechanisms leading to the emergence of “pilgrim” nucleic acids.
References
Chernolovskaya E. L. RNA interference: fighting fire with fire… // Science First Hand, 2008. .No. 1. P. 38–43.
Rykova E. Y. et al. Cell-free and cell-bound circulating nucleic acid complexes: mechanisms of generation, concentration and content, Expert Opin. Biol. Ther., 2012. Suppl. 1. P. 141–153.
Vlassov V. V. Pyshnyi D. V. Vorobjev P. E., Nucleic acids: structures, functions, and applications, in: Handbook of Nucleic Acids Purification, Ed., D. Liu, Boca Raton: CRC Press, 2009.
